How can a quantum wave packet be observed in situ?
Researchers have succeeded in directly observing the free expansion of a single atom's quantum wave packet in continuous space. Using a new imaging technique that combines optical trapping and quantum gas microscopy, they have tracked the spread of the probability density associated with an atom released from its trap with unprecedented precision.
Références
In Situ Imaging of a Single-Atom Wave Packet in Continuous Space, Joris Verstraten et al., Phys. Rev. Lett. 134, 083403– Publié le 28 Février 2025
Doi :10.1103/PhysRevLett.134.083403
Open-access archive : arXiv
Since the work of Louis de Broglie in 1924, the notion of wave-particle duality has been at the heart of quantum mechanics. When an atom is confined in a trap, its wave function is localized as a particle would be, but as soon as it is released (by extinction of the trap), it gradually spreads out in space, according to an evolution predicted by Schrödinger's famous equation. Directly observing the dynamics of such an object in free space is a major experimental challenge, one that would provide access to the fundamental properties of many quantum systems. Until now, experiments on quantum gases with resolution at the level of the individual atom - a technique known as quantum gas microscopy - have been limited to ensembles of atoms evolving in a discrete structure made up of spatially periodic traps, and have not allowed the study of continuous quantum systems.
Ces recherches ont été menées dans les laboratoires suivants
- Laboratoire Kastler Brossel (LKB, CNRS/Collège de France/ENS-PSL/Sorbonne Université)
- Laboratoire collision agrégats réactivité (LCAR, CNRS / Université de Toulouse)
A recent study opens the way to quantum gas microscopy in continuous space. In their experiment, carried out at Laboratoire Kastler Brossel, the researchers first prepared wave packets of individual lithium atoms confined in the potential wells of an optical lattice. Once released in a plane where they could move freely, these atoms were recaptured using a projection method in a deep optical lattice, enabling precise reconstruction of their displacement. By repeating this experiment on a large number of atoms, the researchers were able to reconstruct the spatio-temporal evolution of the wave function with unequalled fidelity. This study establishes a protocol for projecting out an atom from continuous space to the nearest lattice site in a controlled manner, with a fidelity greater than 99%, and lays the foundations for quantum gas microscopy in continuous space. The imaging method developed here can thus be compared to a “CCD sensor” for atomic wave functions.
Beyond this experimental validation, the new method for imaging individual atoms developed by the research group paves the way to the study of more complex quantum systems in continuous space. This imaging method is already being used by the study's researchers to explore strongly correlated fermion systems at the microscopic scale, which are difficult to tackle theoretically. These results are published in the Physical Review Letters.
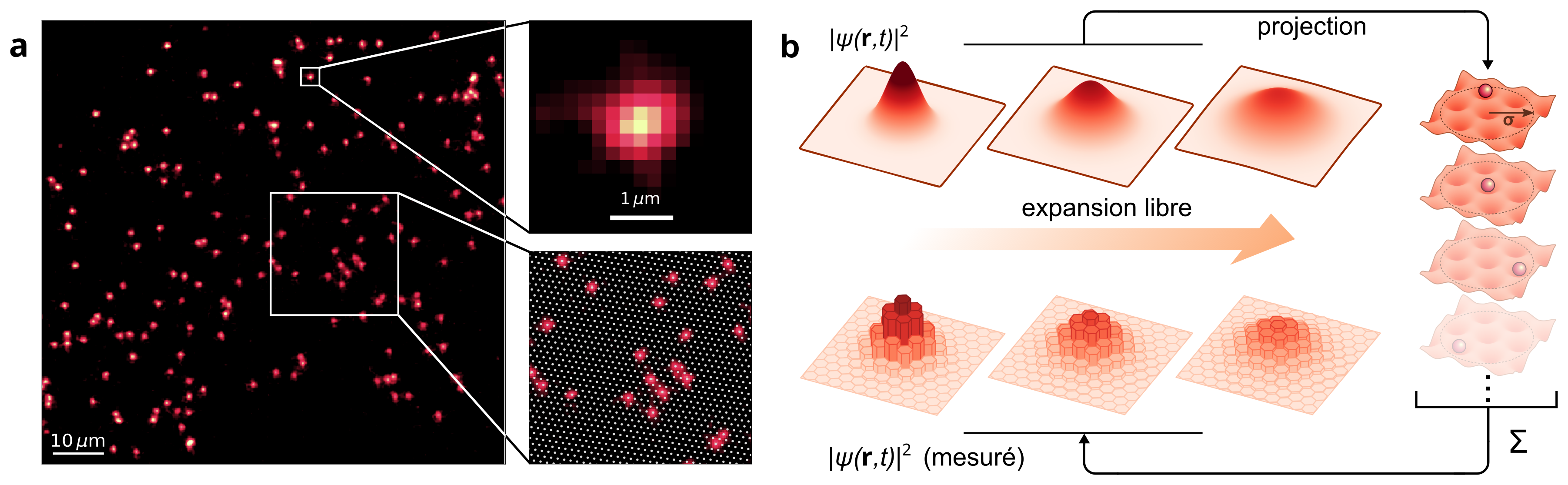